Why Must Ecosystems Receive a Continuous Supply of Energy From the Sun
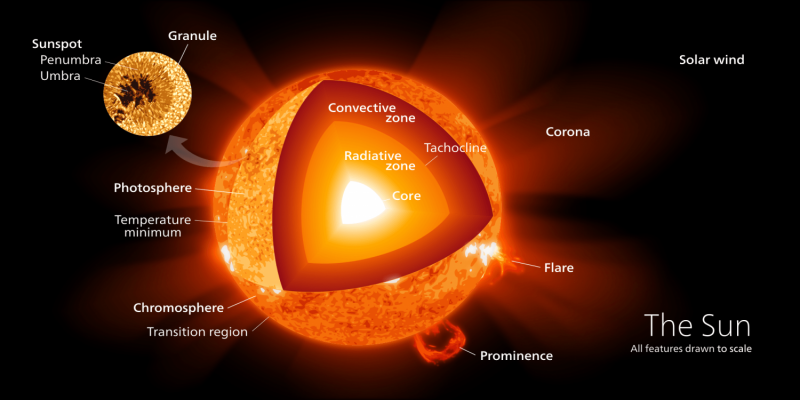
There is a reason life that Earth is the only place in the solar system where life is known to be able to live and thrive. Granted, scientists believe that there may be microbial or even aquatic life forms living beneath the icy surfaces of Europa and Enceladus, or in the methane lakes on Titan. But for the time being, Earth remains the only place that we know of that has all the right conditions for life to exist.
One of the reasons for this is because the Earth lies within our sun's Habitable Zone (aka. "Goldilocks Zone"). This means that it is in right spot (neither too close nor too far) to receive the sun's abundant energy, which includes the light and heat that is essential for chemical reactions. But how exactly does our sun go about producing this energy? What steps are involved, and how does it get to us here on planet Earth?
The simple answer is that the sun, like all stars, is able to create energy because it is essentially a massive fusion reaction. Scientists believe that this began when a huge cloud of gas and particles (i.e. a nebula) collapsed under the force of its own gravity – which is known as Nebula Theory. This not only created the big ball of light at the center of our solar system, it also triggered a process whereby hydrogen, collected in the center, began fusing to create solar energy.
Technically known as nuclear fusion, this process releases an incredible amount of energy in the form of light and heat. But getting that energy from the center of our sun all the way out to planet Earth and beyond involves a couple of crucial steps. In the end, it all comes down to the sun's layers, and the role each of them plays in making sure that solar energy gets to where it can help create and sustain life.
The Core:
The core of the sun is the region that extends from the center to about 20–25% of the solar radius. It is here, in the core, where energy is produced by hydrogen atoms (H) being converted into nuclei of helium (He). This is possible thanks to the extreme pressure and temperature that exists within the core, which are estimated to be the equivalent of 250 billion atmospheres (25.33 trillion KPa) and 15.7 million kelvin, respectively.
The net result is the fusion of four protons (hydrogen nuclei) into one alpha particle – two protons and two neutrons bound together into a particle that is identical to a helium nucleus. Two positrons are released from this process, as well as two neutrinos (which changes two of the protons into neutrons), and energy.
The core is the only part of the sun that produces an appreciable amount of heat through fusion. In fact, 99% of the energy produced by the sun takes place within 24% of the sun's radius. By 30% of the radius, fusion has stopped almost entirely. The rest of the sun is heated by the energy that is transferred from the core through the successive layers, eventually reaching the solar photosphere and escaping into space as sunlight or the kinetic energy of particles.
The sun releases energy at a mass–energy conversion rate of 4.26 million metric tons per second, which produces the equivalent of 384.6 septillion watts (3.846×1026 W). To put that in perspective, this is the equivalent of about 9.192×1010 megatons of TNT per second, or 1,820,000,000 Tsar Bombas – the most powerful thermonuclear bomb ever built!
Radiative Zone:
This is the zone immediately next to the core, which extends out to about 0.7 solar radii. There is no thermal convection in this layer, but solar material in this layer is hot and dense enough that thermal radiation is all that is needed to transfer the intense heat generated in the core outward. Basically, this involves ions of hydrogen and helium emitting photons that travel a short distance before being reabsorbed by other ions.
Temperatures drop in this layer, going from approximately 7 million kelvin closer to the core to 2 million at the boundary with the convective zone. Density also drops in this layer a hundredfold from 0.25 solar radii to the top of the radiative zone, going from 20 g/cm3 closest to the core to just 0.2 g/cm3 at the upper boundary.
Convective Zone:
This is the sun's outer layer, which accounts for everything beyond 70% of the inner solar radius (or from the surface to approx. 200,000 km below). Here, the temperature is lower than in the radiative zone and heavier atoms are not fully ionized. As a result, radiative heat transport is less effective, and the density of the plasma is low enough to allow convective currents to develop.
Because of this, rising thermal cells carry the majority of the heat outward to the sun's photosphere. Once these cells rise to just below the photospheric surface, their material cools, causing their density increases. This forces them to sink to the base of the convection zone again – where they pick up more heat and the convective cycle continues.
At the surface of the sun, the temperature drops to about 5,700 K. The turbulent convection of this layer of the sun is also what causes an effect that produces magnetic north and south poles all over the surface of the sun.
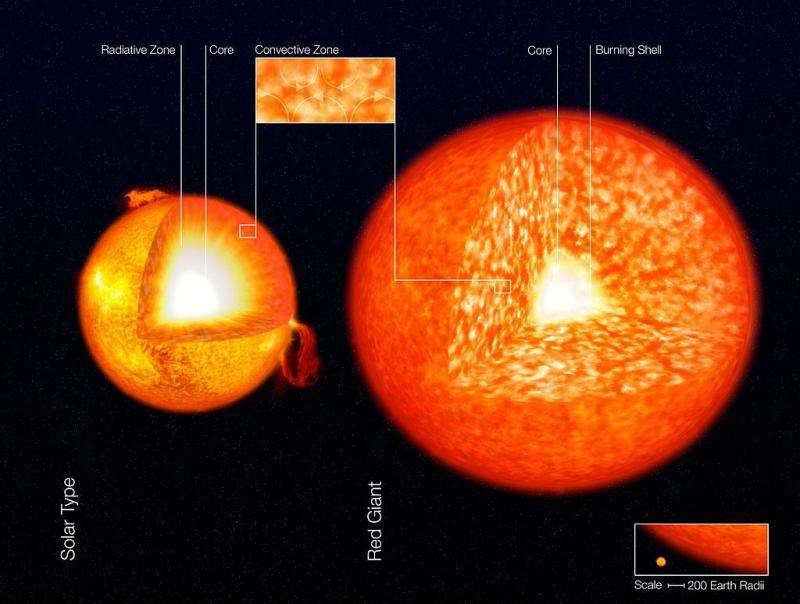
It is also on this layer that sunspots occur, which appear as dark patches compared to the surrounding region. These spots correspond to concentrations in the magnetic flux field that inhibit convection and cause regions on the surface to drop in temperature to compared to the surrounding material.
Photosphere:
Lastly, there is the photosphere, the visible surface of the sun. It is here that the sunlight and heat that are radiated and convected to the surface propagate out into space. Temperatures in the layer range between 4,500 and 6,000 K (4,230 – 5,730 °C; 7646 – 10346 °F). Because the upper part of the photosphere is cooler than the lower part, an image of the sun appears brighter in the center than on the edge or limb of the solar disk, in a phenomenon known as limb darkening.
The photosphere is tens to hundreds of kilometers thick, and is also the region of the sun where it becomes opaque to visible light. The reasons for this is because of the decreasing amount of negatively charged Hydrogen ions (H–), which absorb visible light easily. Conversely, the visible light we see is produced as electrons react with hydrogen atoms to produce H– ions.
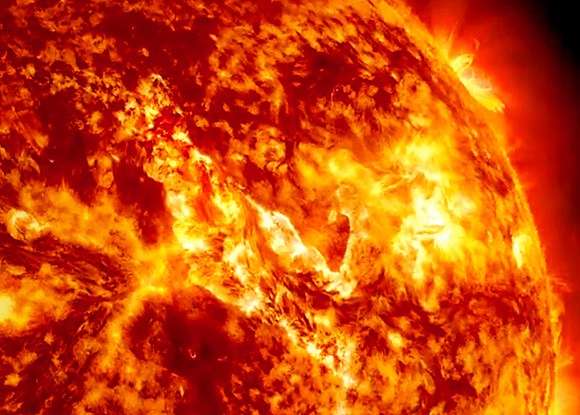
The energy emitted from the photosphere then propagates through space and reaches Earth's atmosphere and the other planets of the solar system. Here on Earth, the upper layer of the atmosphere (the ozone layer) filters much of the sun's ultra-violet (UV) radiation, but passes some onto the surface. The energy that received is then absorbed by the Earth's air and crust, heating our planet and providing organisms with a source of energy.
The sun is at the center of biological and chemical processes here on Earth. Without it, the life cycle of plants and animals would end, the circadian rhythms of all terrestrial creatures would be disrupted; and in time, all life on Earth would cease to exist. The sun's importance has been recognized since prehistoric times, with many cultures viewing it as a deity (more often than not, as the chief deity in their pantheons).
But it is only in the past few centuries that the processes that power the sun have come to be understood. Thanks to ongoing research by physicists, astronomers and biologists, we are now able to grasp how the sun goes about producing energy, and how it passes that on to our solar system. The study of the known universe, with its diversity of star systems and exoplanets – has also helped us to draw comparisons with other types of stars.
Citation: How does the sun produce energy? (2015, December 14) retrieved 22 October 2022 from https://phys.org/news/2015-12-sun-energy.html
This document is subject to copyright. Apart from any fair dealing for the purpose of private study or research, no part may be reproduced without the written permission. The content is provided for information purposes only.
Source: https://phys.org/news/2015-12-sun-energy.html
0 Response to "Why Must Ecosystems Receive a Continuous Supply of Energy From the Sun"
Post a Comment